The internal combustion engine is an engine in which the combustion of a fuel occurs with an oxidizer (usually air) in a combustion chamber. In an internal combustion engine the expansion of the high temperature and pressure gases, which are produced by the combustion, directly applies force to a movable component of the engine, such as the pistons or turbine blades and by moving it over a distance, generate useful mechanical energy.
The term internal combustion engine usually refers to an engine in which combustion is intermittent, such as the more familiar four-stroke and two-stroke piston engines, along with variants, such as the Wankel rotary engine.
The internal combustion engine (or ICE) is quite different from external combustion engines, such as steam or Stirling engines, in which the energy is delivered to a working fluid not consisting of, mixed with or contaminated by combustion products. Working fluids can be air, hot water, pressurised water or even liquid sodium, heated in some kind of boiler by fossil fuel, wood-burning, nuclear, solar etc.
A large number of different designs for ICEs have been developed and built, with a variety of different strengths and weaknesses. Powered by an energy-dense fuel (which is very frequently petrol, a liquid derived from fossil fuels) the ICE delivers an excellent power-to-weight ratio with few safety or other disadvantages. While there have been and still are many stationary applications, the real strength of internal combustion engines is in mobile applications and they dominate as a power supply for cars, aircraft, and boats, from the smallest to the biggest. Only for hand-held power tools do they share part of the market with battery powered devices.
Applications
Internal combustion engines are most commonly used for mobile propulsion in vehicles and portable machinery. In mobile equipment, internal combustion is advantageous since it can provide high power-to-weight ratios together with excellent fuel energy density. Generally using fossil fuel (mainly petroleum), these engines have appeared in transport in almost all vehicles (automobiles, trucks, motorcycles, boats, and in a wide variety of aircraft and locomotives).
Classification
At one time the word, "Engine" (from Latin, via Old French, ingenium, "ability") meant any piece of machinery, a sense that persists in expressions such as siege engine. A "motor" (from Latin motor, "mover") is any machine that produces mechanical power. Traditionally, electric motors are not referred to as, "Engines", however, combustion engines are often referred to as, "motors."
Engines can be classified in many different ways. By the engine cycle used, the layout of the engine, source of energy, the use of the engine, or by the cooling system employed.
Principles Of Operation
Reciprocating:
Two-stroke cycle
Four-stroke cycle
Six stroke engine
Diesel engine
Atkinson cycle
Rotary:
Wankel engine
Four Stroke Configuration
As their name implies, operation of a four stroke internal combustion engines have 4 basic steps that repeat with every two revolutions of the engine:
1: Intake. Combustible mixtures are emplaced in the combustion chamber
2: Compression. The mixtures are placed under pressure
3: Combustion/Expansion. The mixture is burnt, almost invariably a deflagration, although a few systems involve detonation. The hot mixture is expanded, pressing on and moving parts of the engine and performing useful work.
4: Exhaust. The cooled combustion products are exhausted into the atmosphere
Combustion
All internal combustion engines depend on the exothermic chemical process of combustion, the reaction of a fuel, typically with oxygen from the air (though it is possible to inject nitrous oxide in order to do more of the same thing and gain a power boost). The combustion process typically results in the production of a great quantity of heat, as well as the production of steam and carbon dioxide and other chemicals at very high temperature; the temperature reached is determined by the chemical makeup of the fuel and oxidisers.
The most common modern fuels are made up of hydrocarbons and are derived mostly from fossil fuels (petroleum). Fossil fuels include diesel fuel, gasoline and petroleum gas, and the rarer use of propane. Except for the fuel delivery components, most internal combustion engines that are designed for gasoline use can run on natural gas or liquefied petroleum gases without major modifications. Large diesels can run with air mixed with gases and a pilot diesel fuel ignition injection. Liquid and gaseous biofuels, such as ethanol and biodiesel (a form of diesel fuel that is produced from crops that yield triglycerides such as soybean oil), can also be used. Some engines with appropriate modifications can also run on hydrogen gas.
Internal combustion engines require ignition of the mixture, either by spark ignition (SI) or compression ignition (CI). Before the invention of reliable electrical methods, hot tube and flame methods were used.
Gasoline Ignition Process
Gasoline engine ignition systems generally rely on a combination of a lead-acid battery and an induction coil to provide a high-voltage electrical spark to ignite the air-fuel mix in the engine's cylinders. This battery is recharged during operation using an electricity-generating device such as an alternator or generator driven by the engine. Gasoline engines take in a mixture of air and gasoline and compress it to not more than 12.8 bar (1.28 MPa), then use a spark plug to ignite the mixture when it is compressed by the piston head in each cylinder.
Diesel Ignition Process
Diesel engines and HCCI (Homogeneous charge compression ignition) engines, rely solely on heat and pressure created by the engine in its compression process for ignition. The compression level that occurs is usually twice or more than a gasoline engine. Diesel engines will take in air only, and shortly before peak compression, a small quantity of diesel fuel is sprayed into the cylinder via a fuel injector that allows the fuel to instantly ignite. HCCI type engines will take in both air and fuel but continue to rely on an unaided auto-combustion process, due to higher pressures and heat. This is also why diesel and HCCI engines are more susceptible to cold-starting issues, although they will run just as well in cold weather once started. Light duty diesel engines with indirect injection in automobiles and light trucks employ glow plugs that pre-heat the combustion chamber just before starting to reduce no-start conditions in cold weather. Most diesels also have a battery and charging system. Nevertheless, this system is secondary and is added by manufacturers as a luxury for the ease of starting, turning fuel on and off (which can also be done via a switch or mechanical apparatus), and for running auxiliary electrical components and accessories. Most new engines rely on electrical and electronic control system that also control the combustion process to increase efficiency and reduce emissions.
Two Stroke Configuration
Engines based on the two-stroke cycle use two strokes (one up, one down) for every power stroke. Since there are no dedicated intake or exhaust strokes, alternative methods must be used to scavenge the cylinders. The most common method in spark-ignition two-strokes is to use the downward motion of the piston to pressurize fresh charge in the crankcase, which is then blown through the cylinder through ports in the cylinder walls.
Spark-ignition two-strokes are small and light for their power output and mechanically very simple. However, they are also generally less efficient and more polluting than their four-stroke counterparts. In terms of power per cubic centimetre, a single-cylinder small motor application like a two-stroke engine produces much more power than an equivalent four-stroke engine due to the enormous advantage of having one power stroke for every 360 degrees of crankshaft rotation (compared to 720 degrees in a 4 stroke motor).
Small displacement, crankcase-scavenged two-stroke engines have been less fuel-efficient than other types of engines when the fuel is mixed with the air prior to scavenging allowing some of it to escape out of the exhaust port. Modern designs (Sarich and Paggio) use air-assisted fuel injection which avoids this loss, and are more efficient than comparably sized four-stroke engines. Fuel injection is essential for a modern two-stroke engine in order to meet ever more stringent emission standards.
Research continues into improving many aspects of two-stroke motors including direct fuel injection, amongst other things. The initial results have produced motors that are much cleaner burning than their traditional counterparts. Two-stroke engines are widely used in snowmobiles, lawnmowers, string trimmers, chain saws, jet skis, mopeds, outboard motors, and many motorcycles. Two-stroke engines have the advantage of an increased specific power ratio (i.e. power to volume ratio), typically around 1.5 times that of a typical four-stroke engine.
Wankel
The Wankel engine (rotary engine) does not have piston strokes. It operates with the same separation of phases as the four-stroke engine with the phases taking place in separate locations in the engine. In thermodynamic terms it follows the Otto engine cycle, so may be thought of as a "four-phase" engine. While it is true that three power strokes typically occur per rotor revolution due to the 3/1 revolution ratio of the rotor to the eccentric shaft, only one power stroke per shaft revolution actually occurs. This engine provides three power 'strokes' per revolution per rotor giving it a greater power-to-weight ratio than piston engines. This type of engine is most notably used in the current Mazda RX-8, the earlier RX-7, and other models.
Engine Cycle
Two-Stroke
This system manages to pack one power stroke into every two strokes of the piston (up-down). This is achieved by exhausting and re-charging the cylinder simultaneously.
The steps involved here are:
1: Intake and exhaust occur at bottom dead centre. Some form of pressure is needed, either crankcase compression or super-charging.
2: Compression stroke: Fuel-air mix compressed and ignited. In case of Diesel, air compressed, fuel injected and self ignited.
3: Power stroke: Piston is pushed downwards by the hot exhaust gases.
Four-Stroke
Engines based on the four-stroke ("Otto cycle") have one power stroke for every four strokes (up-down-up-down) and employ spark plug ignition. Combustion occurs rapidly, and during combustion the volume varies little ("constant volume"). They are used in cars, larger boats, some motorcycles, and many light aircraft. They are generally quieter, more efficient, and larger than their two-stroke counterparts.
The steps involved here are:
1: Intake stroke: Air and vaporized fuel are drawn in.
2: Compression stroke: Fuel vapour and air are compressed and ignited.
3: Combustion stroke: Fuel combusts and piston is pushed downwards.
4: Exhaust stroke: Exhaust is driven out. During the 1st, 2nd, and 4th stroke the piston is relying on power and the momentum generated by the other pistons. In that case, a four cylinder engine would be less powerful than a six or eight cylinder engine.
There are a number of variations of these cycles, most notably the Atkinson and Miller cycles. The diesel cycle is somewhat different.
Diesel Cycle
Most truck and automotive diesel engines use a cycle reminiscent of a four-stroke cycle, but with a compression heating ignition system, rather than needing a separate ignition system. This variation is called the diesel cycle. In the diesel cycle, diesel fuel is injected directly into the cylinder so that combustion occurs at constant pressure, as the piston moves, rather than with the four stroke with the piston essentially stationary.
Fuels And Oxidizers
Engines are often classified by the fuel (or propellant) used.
Fuels
Nowadays, fuels used include:
Petroleum:
Petroleum spirit (North American term: gasoline, British term: petrol)
Petroleum diesel.
Autogas (liquefied petroleum gas).
Compressed natural gas.
Jet fuel (aviation fuel)
Residual fuel
Vegoils:
Peanut oil and other vegoils.
Biofuels:
Biobutanol (replaces gasoline).
Biodiesel (replaces petrodiesel).
Bioethanol and Biomethanol (wood alcohol) and other biofuels.
Biogas
Even fluidized metal powders and explosives have seen some use. Engines that use gases for fuel are called gas engines and those that use liquid hydrocarbons are called oil engines, however gasoline engines are also often colloquially referred to as, "gas engines" ("petrol engines" in the UK).
The main limitations on fuels are that it must be easily transportable through the fuel system to the combustion chamber, and that the fuel releases sufficient energy in the form of heat upon combustion to make practical use of the engine.
Diesel engines are generally heavier, noisier, and more powerful at lower speeds than gasoline engines. They are also more fuel-efficient in most circumstances and are used in heavy road vehicles, some automobiles (increasingly so for their increased fuel efficiency over gasoline engines), ships, railway locomotives, and light aircraft. Gasoline engines are used in most other road vehicles including most cars, motorcycles, and mopeds. Note that in Europe, sophisticated diesel-engined cars have taken over about 40% of the market since the 1990s. There are also engines that run on hydrogen, methanol, ethanol, liquefied petroleum gas (LPG), and biodiesel. Paraffin and tractor vaporizing oil (TVO) engines are no longer seen.
Hydrogen
At present, hydrogen is mostly used as fuel for rocket engines. In the future, hydrogen might replace more conventional fuels in traditional internal combustion engines. If hydrogen fuel cell technology becomes widespread, then the use of internal combustion engines may be phased out.
Although there are multiple ways of producing free hydrogen, those methods require converting combustible molecules into hydrogen or consuming electric energy. Unless that electricity is produced from a renewable source, and is not required for other purposes, hydrogen does not solve any energy crisis. In many situations the disadvantage of hydrogen, relative to carbon fuels, is its storage. Liquid hydrogen has extremely low density (14 times lower than water) and requires extensive insulation, whilst gaseous hydrogen requires heavy tankage. Even when liquefied, hydrogen has a higher specific energy but the volumetric energetic storage is still roughly five times lower than petrol. However the energy density of hydrogen is considerably higher than that of electric batteries, making it a serious contender as an energy carrier to replace fossil fuels. The 'Hydrogen on Demand' process creates hydrogen as it is needed, but has other issues such as the high price of the sodium borohydride which is the raw material.
Oxidizers
Since air is plentiful at the surface of the earth, the oxidizer is typically atmospheric oxygen which has the advantage of not being stored within the vehicle, increasing the power-to-weight and power to volume ratios. There are other materials that are used for special purposes, often to increase power output or to allow operation under water or in space.
Nitromethane is added to some racing and model fuels to increase power and control combustion.
Nitrous oxide has been used with extra gasoline in specially equipped cars to allow short bursts of added power from engines that otherwise run on gasoline and air.
Other chemicals such as chlorine or fluorine have been used experimentally, but have not been found to be practical.
Engine Capacity
For piston engines, an engine's capacity is the engine displacement, in other words the volume swept by all the pistons of an engine in a single movement. It is generally measured in litres (L) or cubic inches (cu in or in³) for larger engines, and cubic centimetres (abbreviated cc) for smaller engines. Engines with greater capacities are usually more powerful and provide greater torque at lower rpm, but also consume more fuel. Apart from designing an engine with more cylinders, there are two ways to increase an engines' capacity. The first is to lengthen the stroke, the second is to increase the pistons' diameter. In either case, it may be necessary to make further adjustments to the fuel intake of the engine to ensure optimum performance.
Common Components
Combustion Chambers
Internal combustion engines can contain any number of combustion chambers (cylinders), with numbers between one and twelve being common, though as many as 36 (Lycoming R-7755) have been used. Having more cylinders in an engine yields two potential benefits. First, the engine can have a larger displacement with smaller individual reciprocating masses, that is, the mass of each piston can be less thus making a smoother-running engine since the engine tends to vibrate as a result of the pistons moving up and down. Doubling the number of the same size cylinders will double the torque and power. The downside to having more pistons is that the engine will tend to weigh more and generate more internal friction as the greater number of pistons rub against the inside of their cylinders. This tends to decrease fuel efficiency and robs the engine of some of its power. For high-performance gasoline engines using current materials and technology such as the engines found in modern automobiles, there seems to be a break-point around 10 or 12 cylinders after which the addition of cylinders becomes an overall detriment to performance and efficiency. Although, exceptions such as the W16 engine from Volkswagen exist.
Most car engines have four to eight cylinders with some high performance cars having ten, twelve or even sixteen, and some very small cars and trucks having two or three. In previous years, some quite large cars such as the DKW and Saab 92, had two-cylinder or two-stroke engines.
Motorcycles commonly have from one to four cylinders, with a few high performance models having six although, some 'novelties' exist with 8, 10, or 12.
Ignition System
The ignition system of an internal combustion engines depends on the type of engine and the fuel used. Petrol engines are typically ignited by a precisely timed spark, and diesel engines by compression heating. Historically, outside flame and hot-tube systems were used, see hot bulb engine.
Spark
The mixture is ignited by an electrical spark from a spark plug, the timing of which is very precisely controlled. Almost all gasoline engines are of this type. Diesel engines timing is precisely controlled by the pressure pump and injector.
Compression
Ignition occurs as the temperature of the fuel/air mixture is taken over its autoignition temperature, due to heat generated by the compression of the air during the compression stroke. The vast majority of compression ignition engines are diesels in which the fuel is mixed with the air after the air has reached ignition temperature. In this case, the timing comes from the fuel injection system. Very small model engines for which simplicity and light weight is more important than fuel costs use easily ignited fuels (a mixture of kerosene, ether, and lubricant) and adjustable compression to control ignition timing for starting and running.
Ignition Timing
For reciprocating engines, the point in the cycle at which the fuel-oxidizer mixture is ignited has a direct effect on the efficiency and output of the ICE. The thermodynamics of the idealized Carnot heat engine tells us that an ICE is most efficient if most of the burning takes place at a high temperature, resulting from compression near top dead centre. The speed of the flame front is directly affected by the compression ratio, fuel mixture temperature, and Octane rating or cetane number of the fuel. Leaner mixtures and lower mixture pressures burn more slowly requiring more advanced ignition timing. It is important to have combustion spread by a thermal flame front (deflagration), not by a shock wave. Combustion propagation by a shock wave is called detonation and, in engines, is also known as pinging or Engine knocking.
So at least in gasoline-burning engines, ignition timing is largely a compromise between an earlier "advanced" spark which gives greater efficiency with high octane fuel and a later "retarded" spark that avoids detonation with the fuel used. For this reason, high-performance diesel automobile proponents such as, Gale Banks, believe that:
“There’s only so far you can go with an air-throttled engine on 91-octane gasoline. In other words, it is the fuel, gasoline, that has become the limiting factor. While turbocharging has been applied to both gasoline and diesel engines, only limited boost can be added to a gasoline engine before the fuel octane level again becomes a problem. With a diesel, boost pressure is essentially unlimited. It is literally possible to run as much boost as the engine will physically stand before breaking apart. Consequently, engine designers have come to realize that diesels are capable of substantially more power and torque than any comparably sized gasoline engine.”
Fuel Systems
Fuels burn faster and more efficiently when they present a large surface area to the oxygen in air. Liquid fuels must be atomized to create a fuel-air mixture, traditionally this was done with a carburettor in petrol engines and with fuel injection in diesel engines. Most modern petrol engines now use fuel injection too - though the technology is quite different. While diesel must be injected at an exact point in that engine cycle, no such precision is needed in a petrol engine. However, the lack of lubricant in petrol means that the injectors themselves must be more sophisticated.
Carburettor
Simpler reciprocating engines continue to use a carburettor to supply fuel into the cylinder. Although carburettor technology in automobiles reached a very high degree of sophistication and precision, from the mid-1980s it lost out on cost and flexibility to fuel injection. Simple forms of carburettor remain in widespread use in small engines such as lawn mowers and more sophisticated forms are still used in small motorcycles.
Fuel Injection
Larger gasoline engines used in automobiles have mostly moved to fuel injection systems (see Gasoline Direct Injection). Diesel engines have always used fuel injection system because the timing of the injection initiates and controls the combustion.
Autogas (LPG) engines use either fuel injection systems or open- or closed-loop carburettors.
Fuel Pump
Most internal combustion engines now require a fuel pump. Diesel engines use an all-mechanical precision pump system that delivers a timed injection direct into the combustion chamber, hence requiring a high delivery pressure to overcome the pressure of the combustion chamber. Petrol fuel injection delivers into the inlet tract at atmospheric pressure (or below) and timing is not involved, these pumps are normally driven electrically. Gas turbine and rocket engines use electrical systems.
Naturally Aspirated Engines
When air is used with piston engines it can simply suck it in as the piston increases the volume of the chamber. However, this gives a maximum of 1 atmosphere of pressure difference across the inlet valves, and at high engine speeds the resulting airflow can limit potential power output.
Superchargers And Turbochargers
A supercharger is a "forced induction" system which uses a compressor powered by the shaft of the engine which forces air through the valves of the engine to achieve higher flow. When these systems are employed the maximum absolute pressure at the inlet valve is typically around 2 times atmospheric pressure or more.
Turbochargers are another type of forced induction system which has its compressor powered by a gas turbine running off the exhaust gases from the engine.
Parts
For a four-stroke engine, key parts of the engine include the crankshaft, connecting rod, one or more camshafts, and valves. For a two-stroke engine, there may simply be an exhaust outlet and fuel inlet instead of a valve system. In both types of engines there are one or more cylinders, and for each cylinder there is a spark plug, a piston, and a crankpin. A single sweep of the cylinder by the piston in an upward or downward motion is known as a stroke. The downward stroke that occurs directly after the air-fuel mix passes from the carburettor or fuel injector to the cylinder (where it is ignited) is also known as a power stroke.
A Wankel engine has a triangular rotor that orbits in an epitrochoidal (figure 8 shape) chamber around an eccentric shaft. The four phases of operation (intake, compression, power, and exhaust) take place in what is effectively a moving, variable-volume chamber.
Valves
All four-stroke internal combustion engines employ valves to control the admittance of fuel and air into the combustion chamber. Two-stroke engines use ports in the cylinder bore, covered and uncovered by the piston, though there have been variations such as exhaust valves.
Piston Engine Valves
In piston engines, the valves are grouped into 'inlet valves' which admit the entrance of fuel and air and 'outlet valves' which allow the exhaust gases to escape. Each valve opens once per cycle and the ones that are subject to extreme accelerations are held closed by springs that are typically opened by rods running on a camshaft rotating with the engines' crankshaft.
Exhaust Systems
Internal combustion engines have to manage the exhaust of the cooled combustion gas from the engine. The exhaust system frequently contains devices to control pollution, both chemical and noise pollution. In addition, for cyclic combustion engines the exhaust system is frequently tuned to improve emptying of the combustion chamber.
Cooling Systems
Combustion generates a great deal of heat, and some of this transfers to the walls of the engine. Failure will occur if the body of the engine is allowed to reach too high a temperature; either the engine will physically fail, or any lubricants used will degrade to the point that they no longer protect the engine.
Cooling systems usually employ air (air cooled) or liquid (usually water) cooling while some very hot engines using radiative cooling.
Piston
A piston is a component of reciprocating engines. It is located in a cylinder and is made gas-tight by piston rings. Its purpose is to transfer force from expanding gas in the cylinder to the crankshaft via a piston rod and/or connecting rod. In two-stroke engines the piston also acts as a valve by covering and uncovering ports in the cylinder wall.
Crankshaft
Most reciprocating internal combustion engines end up turning a shaft. This means that the linear motion of a piston must be converted into rotation. This is typically achieved by a crankshaft.
Flywheel
The flywheel is a disk or wheel attached to the crank, forming an inertial mass that stores rotational energy. In engines with only a single cylinder the flywheel is essential to carry energy over from the power stroke into a subsequent compression stroke. Flywheels are present in most reciprocating engines to smooth out the power delivery over each rotation of the crank and in most automotive engines also mount a gear ring for a starter. The rotational inertia of the flywheel also allows a much slower minimum unloaded speed and also improves the smoothness at idle. The flywheel may also perform a part of the balancing of the system and so by itself be out of balance, although most engines will use a neutral balance for the flywheel, enabling it to be balanced in a separate operation. The flywheel is also used as a mounting for the clutch or a torque converter in most automotive applications.
Starter Systems
All internal combustion engines require some form of system to get them into operation. Most piston engines use a starter motor powered by the same battery as runs the rest of the electric systems. Small internal combustion engines are often started by pull cords. Motorcycles of all sizes were traditionally kick-started, though all but the smallest are now electric-start. Jump starting refers to assistance from another battery (typically when the fitted battery is discharged), while bump starting refers to an alternative method of starting by the application of some external force, e.g. rolling down a hill.
Lubrication Systems
Internal combustions engines require lubrication in operation that moving parts slide smoothly over each other. Insufficient lubrication subjects the parts of the engine to metal-to-metal contact, friction, heat build-up, rapid wear often culminating in parts becoming friction welded together e.g. pistons in their cylinders. Big end bearings seizing up will sometimes lead to a connecting rod breaking and poking out through the crankcase.
Several different types of lubrication systems are used. Simple two-stroke engines are lubricated by oil mixed into the fuel or injected into the induction stream as a spray. As engines were adapted for automotive use, the need for a high power-to-weight ratio led to increased speeds, higher temperatures, and greater pressure on bearings which in turn required pressure-lubrication for crank bearings and connecting-rod journals. This was provided either by a direct lubrication from a pump, or indirectly by a jet of oil directed at pickup cups on the connecting rod ends which had the advantage of providing higher pressures as the engine speed increased.
Control Systems
Most engines require one or more systems to start and shutdown the engine and to control parameters such as the power, speed, torque, pollution, combustion temperature, efficiency and to stabilise the engine from modes of operation that may induce self-damage such as pre-ignition. Such systems may be referred to as engine control units.
Many control systems today are digital, and are frequently termed FADEC (Full Authority Digital Electronic Control) systems.
Diagnostic Systems
Engine On Board Diagnostics (also known as OBD) is a computerized system that allows for electronic diagnosis of a vehicles' powerplant. The first generation, known as OBD1, was introduced 10 years after the U.S. Congress passed the Clean Air Act in 1970 as a way to monitor a vehicles' fuel injection system. OBD2, the second generation of computerized on-board diagnostics, was codified and recommended by the California Air Resource Board in 1994 and became mandatory equipment aboard all vehicles sold in the United States as of 1996.
Measures Of Engine Performance
Engine types vary greatly in a number of different ways:
Energy efficiency
Fuel consumption (brake specific fuel consumption for shaft engines)
Power to weight ratio
Torque curves
Compression ratio
Energy Efficiency
Once ignited and burnt, the combustion products have more available thermal energy than the original compressed fuel-air mixture (which had higher chemical energy). The available energy is manifested as high temperature and pressure that can be translated into work by the engine. In a reciprocating engine, the high-pressure gases inside the cylinders drive the engine's pistons.
Once the available energy has been removed, the remaining hot gases are vented (often by opening a valve or exposing the exhaust outlet) and this allows the piston to return to its previous position (top dead centre, or TDC). The piston can then proceed to the next phase of its cycle, which varies between engines. Any heat that isn't translated into work is normally considered a waste product and is removed from the engine either by an air or liquid cooling system.
Engine efficiency can be discussed in a number of ways but it usually involves a comparison of the total chemical energy in the fuels, and the useful energy extracted from the fuels in the form of kinetic energy. The most fundamental and abstract discussion of engine efficiency is the thermodynamic limit for extracting energy from the fuel defined by a thermodynamic cycle. The most comprehensive is the empirical fuel efficiency of the total engine system for accomplishing a desired task; for example, the miles per gallon accumulated.
Internal combustion engines are primarily heat engines and as such the phenomenon that limits their efficiency is described by thermodynamic cycles. None of these cycles exceed the limit defined by the Carnot cycle which states that the overall efficiency is dictated by the difference between the lower and upper operating temperatures of the engine. A terrestrial engine is usually and fundamentally limited by the upper thermal stability derived from the material used to make up the engine. All metals and alloys eventually melt or decompose and there is significant researching into ceramic materials that can be made with higher thermal stabilities and desirable structural properties. Higher thermal stability allows for greater temperature difference between the lower and upper operating temperatures, thus greater thermodynamic efficiency.
The thermodynamic limits assume that the engine is operating in ideal conditions, a frictionless world, ideal gases, perfect insulators, and operation at infinite time. The real world is substantially more complex and all the complexities reduce the efficiency. In addition, real engines run best at specific loads and rates as described by their power curve. For example, a car cruising on a highway is usually operating significantly below its ideal load, because the engine is designed for the higher loads desired for rapid acceleration. The applications of engines are used as contributed drag on the total system reducing overall efficiency, such as wind resistance designs for vehicles. These and many other losses result in an engines' real-world fuel economy that is usually measured in the units of miles per gallon (or fuel consumption in litres per 100 kilometres) for automobiles. The miles in miles per gallon represents a meaningful amount of work and the volume of hydrocarbon implies a standard energy content.
Most steel engines have a thermodynamic limit of 37%. Even when aided with turbochargers and stock efficiency aids, most engines retain an average efficiency of about 18%-20%.
There are many inventions concerned with increasing the efficiency of IC engines. In general, practical engines are always compromised by trade-offs between different properties such as efficiency, weight, power, heat, response, exhaust emissions, or noise. Sometimes economy also plays a role in not only the cost of manufacturing the engine itself, but also manufacturing and distributing the fuel. Increasing the engines' efficiency brings better fuel economy but only if the fuel cost per energy content is the same.
Measures Of Fuel Efficiency
For stationary and shaft engines including propeller engines, fuel consumption is measured by calculating the brake specific fuel consumption which measures the number of pounds of fuel that is needed to generate an hours' worth of horsepower-energy. In metric units, the number of grams of fuel needed to generate a kilowatt-hour of energy is calculated.
Internal combustion engines such as reciprocating internal combustion engines produce air pollution emissions, due to incomplete combustion of carbonaceous fuel. The main derivatives of the process are carbon dioxide CO2, water and some soot, also called particulate matter (PM). The effects of inhaling particulate matter have been studied in humans and animals and include asthma, lung cancer, cardiovascular issues, and premature death. There are however some additional products of the combustion process that include nitrogen oxides and sulphur and some uncombusted hydrocarbons, depending on the operating conditions and the fuel-air ratio.
Not all of the fuel will be completely consumed by the combustion process. A small amount of fuel will be present after combustion, some of which can react to form oxygenates, such as formaldehyde or acetaldehyde, or hydrocarbons not initially present in the fuel mixture. The primary causes of this is the need to operate near the stoichiometric ratio for gasoline engines in order to achieve combustion and the resulting "quench" of the flame by the relatively cool cylinder walls, otherwise the fuel would burn more completely in excess air. When running at lower speeds, quenching is commonly observed in diesel (compression ignition) engines that run on natural gas. It reduces the efficiency and increases knocking, sometimes causing the engine to stall. Increasing the amount of air in the engine reduces the amount of the first two pollutants, but tends to encourage the oxygen and nitrogen in the air to combine to produce nitrogen oxides (NOx) that has been demonstrated to be hazardous to both plant and animal health. Further chemicals released are benzene and 1,3-butadiene that are also particularly harmful and not all of the fuel burns up completely, so carbon monoxide (CO) is also produced.
Carbon fuels contain sulphur and impurities that eventually lead to producing sulphur oxides (SO) and sulphur dioxide (SO2) in the exhaust which promotes acid rain. One final element in exhaust pollution is ozone (O3). This is not emitted directly but made in the air by the action of sunlight on other pollutants to form "ground level ozone", which, unlike the "ozone layer" in the high atmosphere, is regarded as a bad thing if the levels are too high. Ozone is broken down by nitrogen oxides, so one tends to be lower where the other is higher.
For the pollutants described above (nitrogen oxides, carbon monoxide, sulphur dioxide, and ozone) there are accepted levels that are set by legislation to which no harmful effects are observed—even in sensitive population groups. For the other three, benzene, 1,3-butadiene, and particulates, there is no way of proving they are safe at any level so the experts set standards where the risk to health is, "exceedingly small".
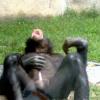
Engine Principles Explained
Started by 2LV8ETR, Oct 12 2009 03:29 PM
#1
Posted 12 October 2009 - 03:29 PM
